Common terminology
It is important to understand some terminology when learning about invasive ventilation. This is by no means an exhaustive list.
- Compliance (L/cm H2O): change in volume / change in pressure.
- A poorly-compliant lung will require a higher distending pressure to achieve a given increase in its volume.
- Dead Space: the proportion of air that is inhaled but does not take part in gas exchange. This can be because no alveoli are present (trachea and bronchioles) or the alveoli are ventilated but not perfused.
- Driving Pressure: This is Pplat – PEEP. High driving pressures increase risk of ventilator associated lung injury.
- Functional Residual Capacity (FRC): the volume of gas remaining in the lungs at end expiration.
- Plateau Pressure (Pplat) vs Peak Inspiratory Pressure (PIP):
- Pplat is the pressure delivered to the alveoli
- Can be determined with an inspiratory hold manoeuvre
- This stops the ventilator transitioning to expiration so the pressure is recorded after an inspiratory breath is completed so there is no flow of gas from the ventilator
- This allows pressure to equilibrate across the respiratory system (from the alveoli to the ETT)
- PIP is the highest pressure the ventilator records during inspiration. It measures the maximal pressure required to drive the air anyway from the ventilator to the alveoli.
- PIP may be significantly greater than Pplat if there is increased airway resistance (i.e. patient biting tube, kinked tube, bronchoconstriction) rather than poor lung compliance.
- High Pplat can increase risk of ventilator associated lung injury.
- Pplat is the pressure delivered to the alveoli
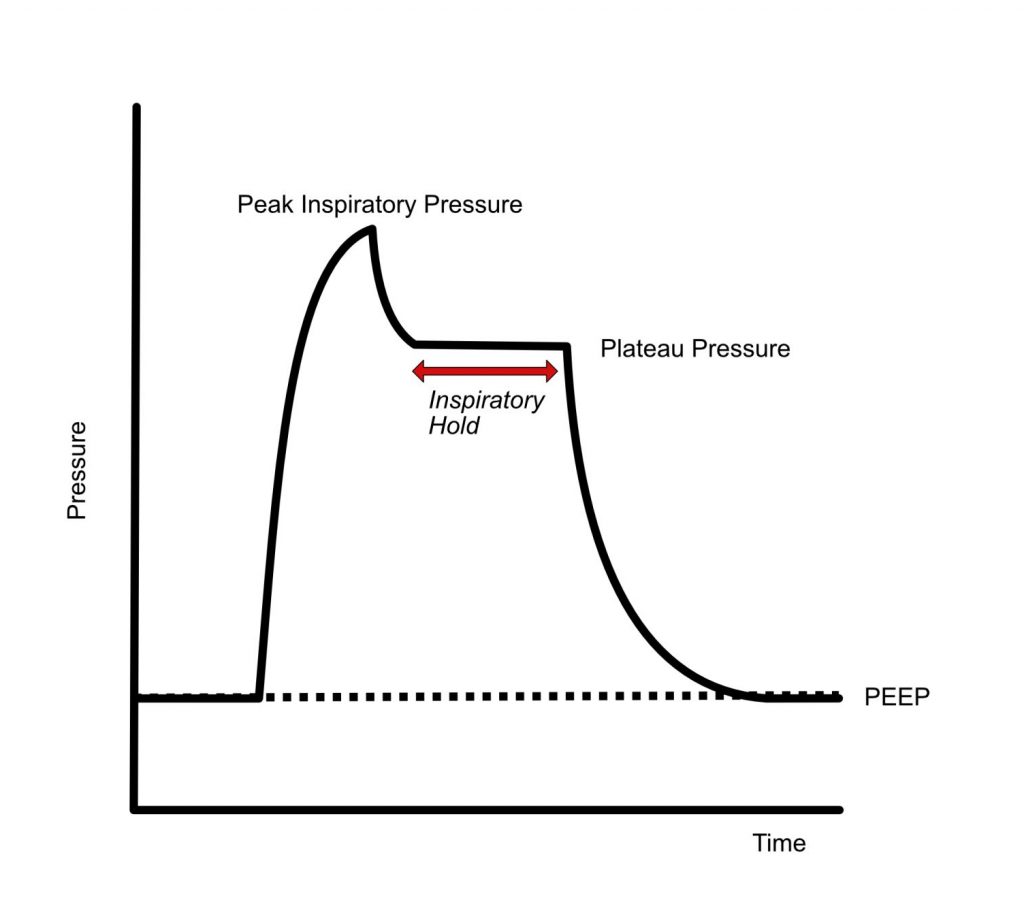
- Positive End Expiratory Pressure (PEEP): the airway pressure at the end of expiration. Intrinsic PEEP (PEEPi) is generated by the patient and occurs when there is an inadequate expiratory time and results in accumulation of gas in the lung (dynamic hyperinflation).
- Shunt: this occurs when alveoli are perfused but not ventilated. Therefore the de- oxygenated haemoglobin is unable to bind with O2. When this blood reaches the arterial system, it “dilutes” the saturated arterial blood, causing a reduction in oxygen concentration. True shunt can not be treated by increasing the FiO2.
- Tidal Volume: the normal volume of air inhaled (and exhaled) in a normal breath.
- Ventilator Associated Lung Injury (VALI): a spectrum of processes that consists of:
- Volutrauma – large tidal volumes cause large changes in volume of alveoli over the respiratory cycle. This causes damage and the release of proinflammatory cytokines which can result in ARDS.
- Barotrauma – increased pressures cause disruption to basement membranes and can lead to pneumothorax
- Atelectotrauma – repeated opening and closing of alveoli can cause release of pro-inflammatory cytokines
- Oxygen Toxicity – locally corrosive to alveoli if used at high concentrations for a prolonged period of time. One approach is to use the lowest amount of O2 required to maintain SpO2 90-92%
- Ventilator associated pneumonia is not classically a VALI but it has been included for awareness
The physiology of invasive ventilation
Invasive ventilation does not reflect the normal physiology of spontaneous ventilation. This involves the generation of negative pressures, which creates a pressure gradient down which gas can flow into the alveoli.
The fundamental change with invasive ventilation is that it relies on positive pressure created by the ventilator to drive gas flow.
Benefits of positive pressure ventilation (PPV) include:
- Alveolar recruitment (the positive pressure can re-open closed alveoli). A greater number of open alveoli increases FRC and improves oxygenation.
- Decreased work of breathing – as more of the alveoli remain open therefore less work is required to keep re-opening closed alveoli.
- Reduced airway resistance as recruited alveoli keep smaller airways open.
- Improvement in lung compliance.
Positive pressure ventilation can also cause deleterious effects. These include:
- Respiratory
- There may be alterations in dead space, especially increased dead space at high PEEP levels.
- Ventilator Associated Lung Injury
- Both VALI and positive pressure ventilation can affect the production and function of surfactant.
- Cardiovascular
- Generally PPV decreases cardiac output (CO) and mean arterial pressure by decreasing venous return due to increased (more positive) intrathoracic pressure. This reduction in CO increases as PEEP increases.
- In some patients with impaired left ventricular (LV) function, the increase in intrathoracic pressure will reduce LV afterload and increase CO.
- Effect on pulmonary vascular resistance (PVR) is variable and dependent on lung volumes and amount of PEEP applied. Increased PVR can significantly impair right ventricular function.
- Generally PPV decreases cardiac output (CO) and mean arterial pressure by decreasing venous return due to increased (more positive) intrathoracic pressure. This reduction in CO increases as PEEP increases.
Settings used in Mechanical Ventilation
Ventilator modes
Simply, ventilators are designed to drive a flow of a gas into the alveoli. A ventilated breath can be broken down into 4 parts:
- Trigger
- Flow
- Cycling
- Expiration
- What is the trigger for the breath?
- Ventilator Driven (Mandatory):
- Time – if set to 12 bpm then ventilator will deliver a breath every 5 seconds. This forms the basis for mandatory breaths (entirely ventilator driven).
- Patient Driven (Spontaneous):
- Flow – the most comfortable trigger. The ventilator detects that the patient has tried to initiate a breath by detecting changes in flow. Normally, a ventilator has a continuous flow in the inspiratory and expiratory limb of the ventilator circuit. If a patient wants to take a breath, some of that flow will “disappear” into the lungs. If this is greater than the trigger setting (normally 1-2L/min) this will result in a supported breath being given. It can be oversensitive (i.e. a breath delivered when the patient didn’t want it).
- Pressure – the patient inhales against a closed inspiratory valve. The ventilator detects this and delivers a supported breath.
- These form the basis for supported modes of ventilation, in which the patient initiates the breath but the ventilator then takes over. This results in better patient comfort as it allows the patient to breathe when they wish.
- Ventilator Driven (Mandatory):
- What will control or limit ventilator flow?
- Volume – a pre-set tidal volume is delivered and can not be exceeded. This is usually given at a given flow rate which cannot be exceeded (flow limited).
- This results in typical “square wave” flow – time waveform
- Note that the machine will deliver the volume at any pressure, so PIP (and Pplat) must be monitored. In poorly compliant lungs the pressure required to deliver this set volume may be too high.
- Pressure – a pre-set pressure is delivered and cannot be exceeded.
- This results in typical “square wave” pressure – time waveform
- If lungs are poorly compliant, then the tidal volume delivered for a given pressure may be inadequate, so tidal volumes must be monitored.
- Volume – a pre-set tidal volume is delivered and can not be exceeded. This is usually given at a given flow rate which cannot be exceeded (flow limited).
- How does the ventilator know when to transition to expiration (cycling)?
- Time cycling – when the set inspiratory time has elapsed
- Flow cycling – such as in pressure support (see below)
- Other cycling methods include:
- Pressure
- Volume
- What happens in expiration?
- Expiration is generally passive
- PEEP is the one variable that can be altered in the expiratory phase.
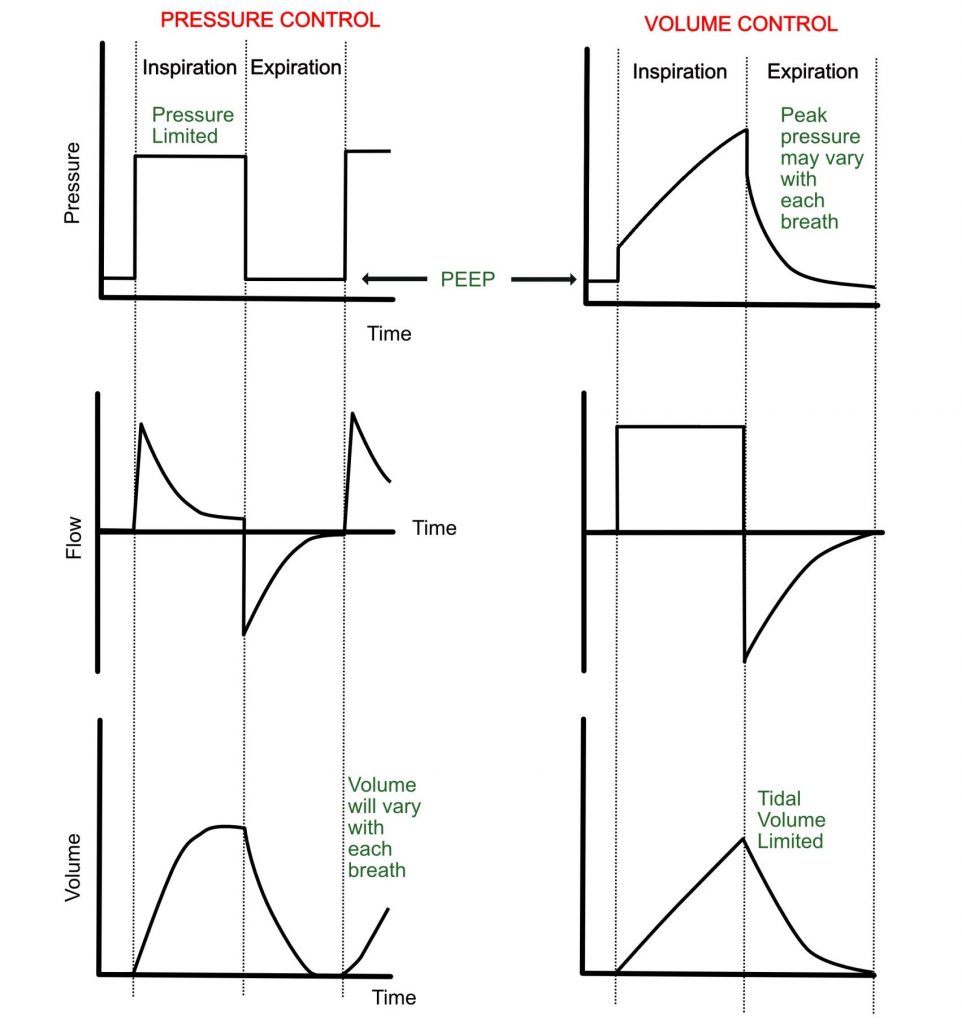
Some common modes
Continuous mandatory ventilation
- Volume Control (VC) and Pressure Control (PC) modes are mandatory, time cycled volume or pressure limited breaths.
SIMV (Synchronised Intermittent Mandatory Ventilation) + PS (Pressure Support)
- This mode delivers a minimum number of breaths whilst also allowing spontaneous breaths.
- This is easier to explain with an example:
- The programmed settings are: 12 breaths x 500mls tidal volume and pressure support is 10 cm H20.
- If the ventilated patient has sufficient respiratory drive to have a respiratory rate of 20/min then:
- 12 of these breaths will be volume controlled (500mls), and these will be synchronised to the respiratory effort of the patient.
- 8 of these breaths will be pressure supported
- If insufficient drive (RR < 12) some of the breaths will be time triggered, mandatory breaths.
Pressure support (PS)
- The ventilator will support spontaneously triggered breath with a certain amount of pressure.
- This is maintained until the inspiratory flow falls to a certain value (e.g. 25% of the inspiratory peak flow) and the ventilator will cycle to expiration.
- Ventilators have a back-up apnoea mandatory ventilatory mode in case the patient’s respiratory drive is inadequate.
APRV (Airway Pressure Release Ventilation)
- This is a ventilator strategy that uses inverse ratios (inspiratory time > expiratory time).
- In APRV, a pressure (PHigh) is set and delivered for a certain period of time (THigh).
- Releases occur for a given time (TLow) and this usually has a pressure set to 0 cmH2O (PLow). The number of releases will vary depending on the need to eliminate CO2. The setting of TLow is complicated and should be done by someone experienced in the use of APRV.
- Despite a PLow often being set to 0 cmH2O, the recorded pressure is usually 2-3 cmH2O. This is because the setting of TLow does not allow full expiration, and as such there is some residual positive pressure remaining in the lungs at end expiration.
- The prolonged time at PHigh enables alveolar recruitment and prevention of alveolar collapse. Patients are also free to spontaneously breath during the THigh.
- Special care is required to ensure that delivered tidal volumes are not too large – this could lead to ventilator-associated lung injury.
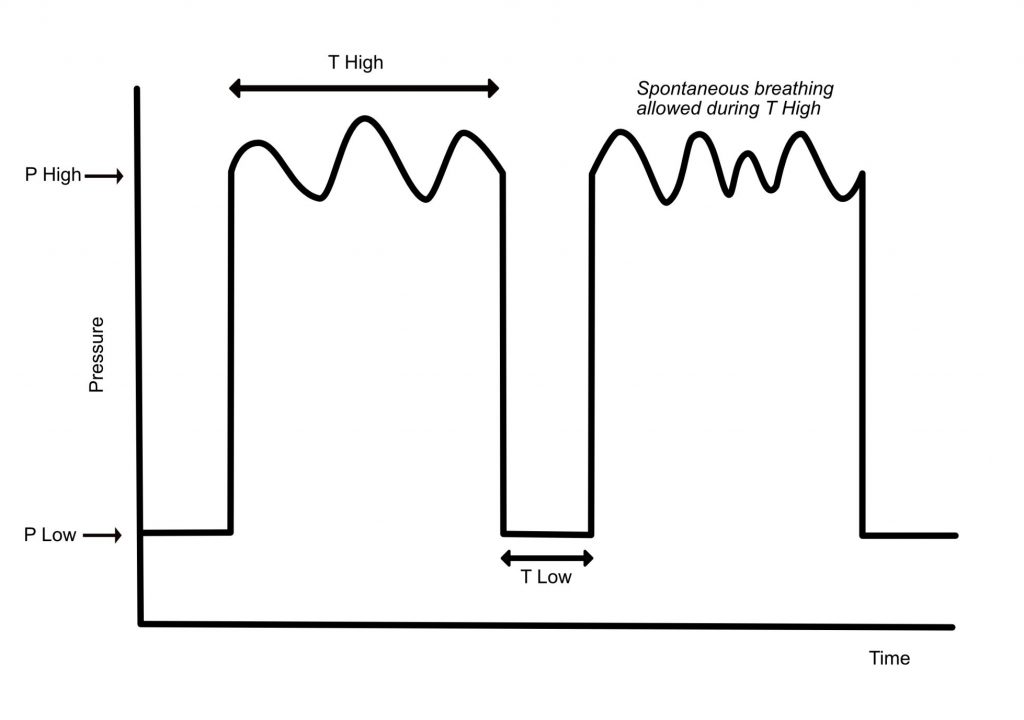
Other settings on a ventilator
- FiO2 – should be set to the lowest level to minimise oxygen toxicity
- Respiratory Rate (RR) – importance for CO2 clearance.
- CO2 clearance is proportional to minute volume (MV = RR x Tidal Volume) – therefore if CO2 climbing this can be treated by increasing tidal volume or RR to increase MV.
- Increasing RR (at higher rates or obstructive lung disease) may compromise expiratory time and lead to dynamic hyperinflation. Permissive hypercapnoea is tolerated in these situations.
- PEEP – usually set to 5 – 10 cm H20. In cases of hypoxia this may be increased.
- I:E Ratio – this is the ratio of inspiratory time to expiratory time. Altering respiratory rate or inspiratory time will alter expiratory time as they are all dependent on each other. Normally, I:E is set around 1:2 (mimics usual breathing).
Special considerations and troubleshooting
Special considerations for the invasively ventilated patient
- Lung Protective Ventilation
- See ARDS section
- Dysynchrony
- Occurs when a ventilator is fighting the patient.
- Problems can result from all parts of the ventilated breath including triggering, flow and cycling.
- Management principles include:
- ABCDE approach – including ensuring patent ventilator circuit.
- May need an increase in sedation +/- muscle relaxant (this may just be temporary while troubleshooting the ventilator to ensure patient safety).
- Try and select a patient triggered mode.
- Review trigger sensitivity. Important to exclude causes of auto-triggering such as cardiac oscillations or patient movement, but sensitive enough that the ventilator delivers a breath when the patient wants.
- Review flow rate – if flow rate low then ventilator may not deliver enough volume if breath is time limited.
- Review flow cycling trigger if appropriate – may need to be increased or decreased.
- Weaning
- The process by which the patient is liberated from mechanical ventilation.
- May be a short or prolonged process and require other ventilatory strategies (e.g. tracheostomy).
- Before weaning the cause for ventilation needs to have resolved, and the patient stable and co-operative to ensure compliance with the weaning process.
- Ventilatory support needs to be reduced (low FiO2 and low PEEP) and patient driven (i.e. they need good neuromuscular function). The patient also needs a good cough and minimal secretion burden.
Troubleshooting the invasively ventilated patient
An approach to acutely hypoxic invasively ventilated patients
- Senior help
- Increase FiO2 to 1.0
- “DOPES”
- Dislodgement of ETT – ETT can often be advanced too far, and cause collapse of the lung not being ventilated
- Obstruction of ETT – kinked tubing, sputum
- Pneumothorax
- Equipment Issues
- Stacked breaths – inadequate expiratory time resulting in dynamic hyperinflation or Shunt.
- Classically (consideration needed in COVID-19 patients) treatment would involve disconnection and using a bag valve mask (BVM) and 100% O2. This removes any equipment issues (which may later require senior help to review).
- If no chest wall rise and hard to BVM then likely issue with tube (or patient).
- Try passing a suction catheter down to remove any sputum and rule out tube obstruction
- Ensure end tidal CO2 confirms endo-tracheal placement of tube
- Ensure no cuff leak can be heard (a “gurgling” noise)
- Patient factors like diffuse bronchospasm may also cause minimal chest rise
- If some chest wall rise the problem more likely with patient
- If asymmetric then consider bronchial intubation, sputum plug or pneumothorax
- Other causes include PE, oedema, infection
- To improve oxygenation:
- Treat suspected cause
- Review the timeline of onset as this may help work out the cause of hypoxia
- Can increase FiO2 as mentioned
- Can increase PEEP to recruit closed alveoli
- Can increase mean airway pressure. This can be done by increasing PEEP or inspiratory time. If inspiratory time is increased, then longer periods of higher pressure are delivered thus increasing the mean pressure. Note, that longer inspiratory times can be uncomfortable for patients.
- Treat suspected cause
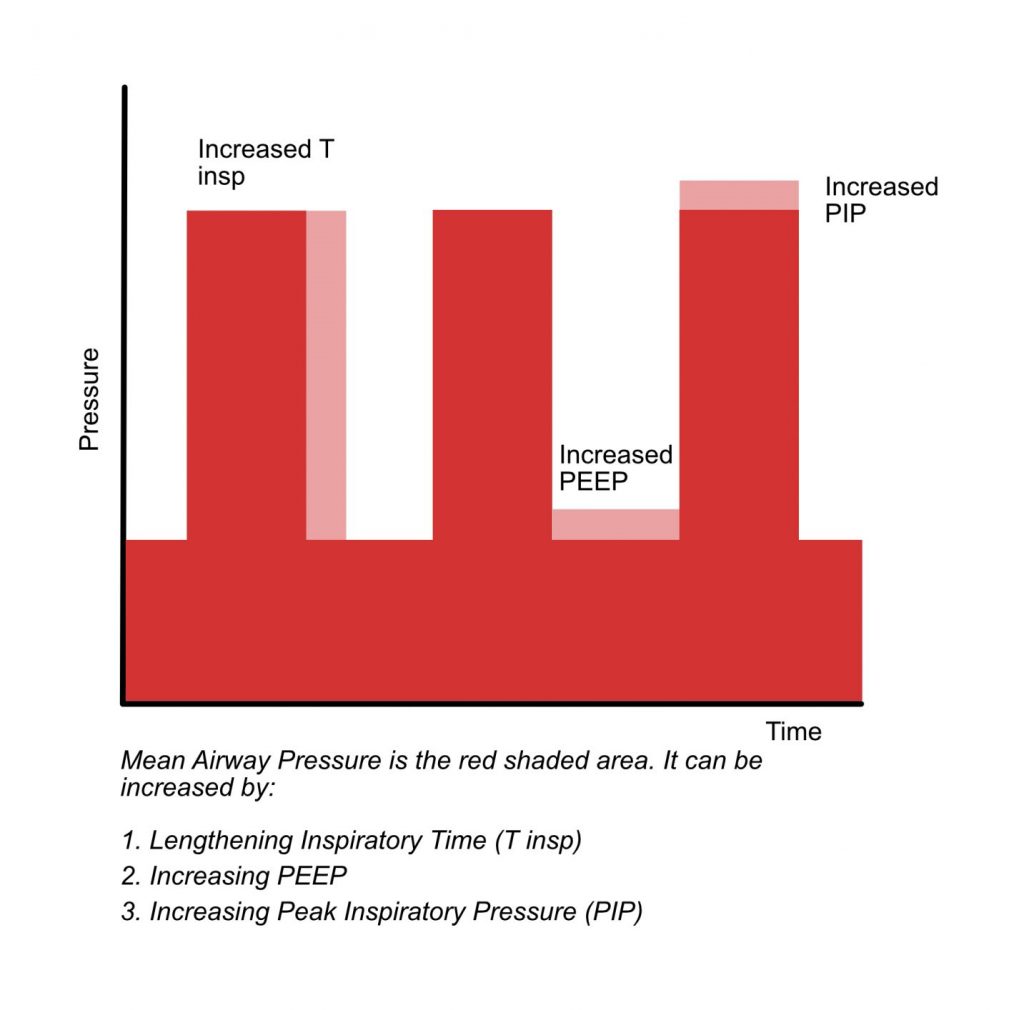
An approach to high peak pressure alarms
- Requires a similar approach to the hypoxic patient.
- Causes include equipment issues (ventilator settings, patient biting, kinked circuit, circuit obstruction) and patient issues.
- In a non-COVID-19 patient, disconnection from the ventilator and manual ventilation via a BVM is important.
- If there is no difficulty manually ventilating the patient, then it is likely that the reason for the high pressure alarm relates to some part of the equipment that has been disconnected.
- If manual ventilation is difficult (or feels “stiff”) then the issue will be from the endotracheal tube onwards.
- It would be important to examine the patient and look for and treat potential causes. These include:
- A CXR may provide useful diagnostic information
- Passing a suction catheter to ensure tube patency and remove any secretions. Large volume secretions can obstruct a bronchus resulting in lobar collapse. This may necessitate bronchoscopy.
- Bronchospasm
- An inspiratory hold manoeuvre can be carried out if the PIP is high. If Pplat is normal then this may suggest bronchospasm.
- Causes of unilateral chest wall movement may include pneumothorax, migration of ETT or lobar collapse
- Dysynchrony
Rescue Strategies
- Proning – the act of turning a ventilated patient onto their front or “prone”. Benefits include improved V/Q mismatch and gas exchange, reduced atelectasis, and improved secretion drainage.
- Inhaled therapies – these include inhaled nitric oxide, or prostaglandins and aim to improve V/Q mismatch.
- APRV (Airway Pressure Release Ventilation) – sometimes used as a rescue method of ventilation in severe respiratory failure.
- ECMO (Extracorporeal Membrane Oxygenation) – in severe respiratory failure a veno-venous configuration is most commonly used which enables blood to be removed from and returned to the venous system near the right atrium. The blood is oxygenated by a mechanical oxygenator in the ECMO circuit instead of the lungs. Mechanical ventilation (usually with very small tidal volumes) will continue to ensure the lungs do not fully de-recruit.
References and Further Reading
RebelEM: Simplifying Mechanical Ventilation
Nepean ICU: Ventilation Cheat Sheet
BASIC Course: Introduction to Mechanical Ventilation
LITFL: Prone position and Mechanical Ventilation
British Journal of Anaesthesia Education
British Journal of Hospital Medicine
- Management of hypoxaemia in the critically ill patient
- Mechanical Ventilation for the non-anaesthetist 1: physiology and mechanics
- Mechanical Ventilation for the non-anaesthetist 2: practical tips
Author: George Walker